1-Sentence-Summary: Turbulent flame speeds of natural gas, methane-propane, and methane-ethane mixtures are explored in small- and large-scale wedge-shaped vessels with obstacles.
Authors: K. van Wingerden, G.H. Pedersen, and B.A. Wilkins
Read in: Three Minutes
Favourite quote from the paper:
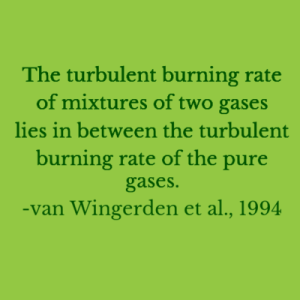
In this short short symposium paper, the authors present experimental turbulent flame propagation results in wedge-shaped vessels with obstacles. Test are performed in a small scale wedge with a length of 1 meter, height of 0.125 meter, and angle of 17 degrees. The five baffle-like obstructions have blockage ratios of 16%, 33%, and 50% by cross-sectional area. The large scale vessel is exactly 10 times the size of the small-scale wedge.
The fuels tested include methane, natural gas, methane-propane mixtures, and methane-ethane mixtures. Flame speed and maximum overpressure are given for different fuel concentrations.
Three of the main findings from this paper are:
- Natural gas could be represented by methane-ethane or methane-propane mixtures.
- Large scale flame speeds and maximum overpressure are higher than in the small-scale vessel.
- Turbulent flame speed increases non-linearly with laminar burning velocity due to feedback between gas expansion and obstacle interaction.
The following sections outline the main findings in more detail. The interested reader is encouraged to view the complete article at the link provided below.
Finding #1: Methane-propane or methane-ethane mixtures can be substituted for natural gas testing
Natural gas flame speeds are compared to mixtures of methane and ethane in the small-scale vessel. Furthermore, natural gas maximum pressures are compared to mixtures of methane and propane in the large-scale vessel. In both cases the mixtures of methane and the second fuel were indistinguishable from the natural gas explosion results. Furthermore, the flame speed in the small-scale vessel was shown to decrease linearly with methane concentration.
Finding #2: Flame speeds and maximum overpressure are higher in the large-scale vessel
The experimental results show that overpresssure in the large-scale chamber is an order higher than in the small scale chamber. The authors state this represents a 5 times increase in flame speed between the vessels. For example, the flame speed in the large scale chamber reaches 250 m/s, where the small scale tests are between 50 – 100 m/s. The authors state that increased flame-folding and hydrodynamic instabilities result in the higher flame speed in the larger vessel.
Finding #3: Turbulent combustion rate increases non-linearly with laminar burning velocity
The authors use a mathematical relationship presented previously in the literature to demonstrate that turbulent flame speed increases in a non-linear manner with laminar flame speed. Starting with the relation proposed by Bray, 1990 based on the experimental data of Abdel-Gayed at al., 1987:
\(S_{T} = 15.0S_{L}^{0.78}u_{T}^{0.412}L_{T}^{0.196}\)
where \(S_{T}\) is the turbulent flame speed, \(S_{L}\) is the laminar flame speed, \(u_{T}\) is the turbulent velocity fluctuation, \(L_{T}\) is the turbulent length scale, and the kinematic viscosity was assumed to be 20 mm2/s.
The authors state that assuming the turbulence intensity in the wake of an obstacle is proportional to the flow speed ahead of the flame (and therefore a function of the density ratio \(\rho_{u}/\rho_{b}\)) the following relation is developed:
\(v_{f} = 88\left(\rho_{u}/\rho{b}\right)^{1.68}p^{1.68}S_{L}^{1.32}L_{T}^{0.32}\)
where \(p\) is a “constant describing the relationship between turbulence intensity in the wake of an obstacle and the main flow speed”, and \(v_{f}\) is the flame speed.
This final relation demonstrates a non-linear increase in the flame speed with laminar burning velocity. This makes sense physically as well, due to coupling effects between flow expansion, flame speed, and feedback between the mean flow and obstacles. Flame folding and hydrodynamic instabilities were also found to be important for flame speeds in the larger wedge-shaped vessel. Based on comparison between these results and the typical effects of flame folding and hydrodynamic instabilities, the combustion relation from Bray, 1990 is justified for inclusion in the FLACS simulation code.
My Personal Take-Aways From
“Turbulent Flame Propagation in Gas Mixtures”
This brief paper demonstrates some interesting points on turbulent flame speeds in different size vessels. For example, the paper demonstrates that turbulent flame speed increases linearly with methane concentration in mixtures with propane gas. These turbulent flames tend to be dominated by transport phenomena which are dependent on the turbulent mixing. Therefore, the role of combustion kinetics is reduced in these systems over ignition studies and laminar flames. The discussion given in the paper on the non-linear increase in burning velocity also reinforces this point.
Full Citation: [bibtex file=references.bib key=VanWingerden1994]